David Robson writes: Some 36-year-olds choose to collect vintage wine, vinyl records or sports memorabilia. For Richard Simcott, it is languages. His itch to learn has led him to study more than 30 foreign tongues – and he’s not ready to give up.
During our conversation in a London restaurant, he reels off sentences in Spanish, Turkish and Icelandic as easily as I can name the pizza and pasta on our menu. He has learned Dutch on the streets of Rotterdam, Czech in Prague and Polish during a house share with some architects. At home, he talks to his wife in fluent Macedonian.
What’s remarkable about Simcott isn’t just the number and diversity of languages he has mastered. It’s his age. Long before grey hairs appear and waistlines expand, the mind’s cogs are meant to seize up, making it difficult to pick up any new skill, be it a language, the flute, or archery. Even if Simcott had primed his mind for new languages while at school, he should have faced a steep decline in his abilities as the years went by – yet he still devours unfamiliar grammars and strange vocabularies to a high level. “My linguistic landscape is always changing,” he says. “If you’re school-aged, or middle-aged – I don’t think there’s a big difference.”
A decade ago, few neuroscientists would have agreed that adults can rival the learning talents of children. But we needn’t be so defeatist. The mature brain, it turns out, is more supple than anyone thought. “The idea that there’s a critical period for learning in childhood is overrated,” says Gary Marcus, a psychologist at New York University. What’s more, we now understand the best techniques to accelerate knowledge and skill acquisition in adults, so can perhaps unveil a few tricks of the trade of super-learners like Simcott. Whatever you want to learn, it’s never too late to charge those grey cells.
The idea that the mind fossilises as it ages is culturally entrenched. The phrase “an old dog will learn no tricks” is recorded in an 18th century book of proverbs and is probably hundreds of years older.
When researchers finally began to investigate the adult brain’s malleability in the 1960s, their results appeared to agree with the saying. Most insights came indirectly from studies of perception, which suggested that an individual’s visual abilities were capped at a young age. For example, restricting young animals’ vision for a few weeks after birth means they will never manage to see normally. The same is true for people born with cataracts or a lazy eye – repair too late, and the brain fails to use the eye properly for life. “For a very long time, it seemed that those constraints were set in stone after that critical period,” says Daphne Bavelier at the University of Rochester, New York.
These are extreme circumstances, of course, but the evidence suggested that the same neural fossilisation would stifle other kinds of learning. Many of the studies looked at language development – particularly in families of immigrants. While the children picked up new tongues with ease, their parents were still stuttering broken sentences. But if there is a critical period for foreign language learning, everyone should be affected equally; Simcott’s ability to master a host of languages should be as impossible as a dog playing the piano. [Continue reading…]
Category Archives: Neuroscience
We’re only beginning to understand how our brains make maps
The Atlantic: About 40 years ago, researchers first began to suspect that we have neurons in our brains called “place cells.” They’re responsible for helping us (rats and humans alike) find our way in the world, navigating the environment with some internal sense of where we are, how far we’ve come, and how to find our way back home. All of this sounds like the work of maps. But our brains do impressively sophisticated mapping work, too, and in ways we never actively notice.
Every time you walk out your front door and past the mailbox, for instance, a neuron in your hippocampus fires as you move through that exact location – next to the mailbox – with a real-world precision down to as little as 30 centimeters. When you come home from work and pass the same spot at night, the neuron fires again, just as it will the next morning. “Each neuron cares for one place,” says Mayank Mehta, a neurophysicist at UCLA. “And it doesn’t care for any other place in the world.”
This is why these neurons are called “place cells.” And, in constantly shuffling patterns, they generate our cognitive maps of the world. Exactly how they do this, though, has remained a bit of an enigma. The latest research from Mehta and his colleagues, published this month in the online edition of the journal Science, provides more clues. It now appears as if all of the sensory cues around us – the smell of a pizzeria, the feel of a sidewalk, the sound of a passing bus – are much more integral to how our brains map our movement through space than scientists previously believed. [Continue reading…]
The secret lives (and deaths) of neurons
UNC School of Medicine: As the human body fine-tunes its neurological wiring, nerve cells often must fix a faulty connection by amputating an axon — the “business end” of the neuron that sends electrical impulses to tissues or other neurons. It is a dance with death, however, because the molecular poison the neuron deploys to sever an axon could, if uncontained, kill the entire cell.
Researchers from the University of North Carolina School of Medicine have uncovered some surprising insights about the process of axon amputation, or “pruning,” in a study published May 21 in the journal Nature Communications. Axon pruning has mystified scientists curious to know how a neuron can unleash a self -destruct mechanism within its axon, but keep it from spreading to the rest of the cell. The researchers’ findings could offer clues about the processes underlying some neurological disorders.
“Aberrant axon pruning is thought to underlie some of the causes for neurodevelopmental disorders, such as schizophrenia and autism,” said Mohanish Deshmukh, PhD, professor of cell biology and physiology at UNC and the study’s senior author. “This study sheds light on some of the mechanisms by which neurons are able to regulate axon pruning.”
Axon pruning is part of normal development and plays a key role in learning and memory. Another important process, apoptosis — the purposeful death of an entire cell — is also crucial because it allows the body to cull broken or incorrectly placed neurons. But both processes have been linked with disease when improperly regulated. [Continue reading…]
Fishing for memories
Riken: In our interaction with our environment we constantly refer to past experiences stored as memories to guide behavioral decisions. But how memories are formed, stored and then retrieved to assist decision-making remains a mystery. By observing whole-brain activity in live zebrafish, researchers from the RIKEN Brain Science Institute have visualized for the first time how information stored as long-term memory in the cerebral cortex is processed to guide behavioral choices.
The study, published today in the journal Neuron, was carried out by Dr. Tazu Aoki and Dr. Hitoshi Okamoto from the Laboratory for Developmental Gene Regulation, a pioneer in the study of how the brain controls behavior in zebrafish.
The mammalian brain is too large to observe the whole neural circuit in action. But using a technique called calcium imaging, Aoki et al. were able to visualize for the first time the activity of the whole zebrafish brain during memory retrieval.
Calcium imaging takes advantage of the fact that calcium ions enter neurons upon neural activation. By introducing a calcium sensitive fluorescent substance in the neural tissue, it becomes possible to trace the calcium influx in neurons and thus visualize neural activity.
The researchers trained transgenic zebrafish expressing a calcium sensitive protein to avoid a mild electric shock using a red LED as cue. By observing the zebrafish brain activity upon presentation of the red LED they were able to visualize the process of remembering the learned avoidance behavior.
They observe spot-like neural activity in the dorsal part of the fish telencephalon, which corresponds to the human cortex, upon presentation of the red LED 24 hours after the training session. No activity is observed when the cue is presented 30 minutes after training.
In another experiment, Aoki et al. show that if this region of the brain is removed, the fish are able to learn the avoidance behavior, remember it short-term, but cannot form any long-term memory of it.
“This indicates that short-term and long-term memories are formed and stored in different parts of the brain. We think that short-term memories must be transferred to the cortical region to be consolidated into long-term memories,” explains Dr. Aoki.
The team then tested whether memories for the best behavioral choices can be modified by new learning. The fish were trained to learn two opposite avoidance behaviors, each associated with a different LED color, blue or red, as a cue. They find that presentation of the different cues leads to the activation of different groups of neurons in the telencephalon, which indicates that different behavioral programs are stored and retrieved by different populations of neurons.
“Using calcium imaging on zebrafish, we were able to visualize an on-going process of memory consolidation for the first time. This approach opens new avenues for research into memory using zebrafish as model organism,” concludes Dr. Okamoto.
Bach to the blues, our emotions match music to colors
>UC Berkeley: Whether we’re listening to Bach or the blues, our brains are wired to make music-color connections depending on how the melodies make us feel, according to new research from the University of California, Berkeley. For instance, Mozart’s jaunty Flute Concerto No. 1 in G major is most often associated with bright yellow and orange, whereas his dour Requiem in D minor is more likely to be linked to dark, bluish gray.
Moreover, people in both the United States and Mexico linked the same pieces of classical orchestral music with the same colors. This suggests that humans share a common emotional palette – when it comes to music and color – that appears to be intuitive and can cross cultural barriers, UC Berkeley researchers said.
“The results were remarkably strong and consistent across individuals and cultures and clearly pointed to the powerful role that emotions play in how the human brain maps from hearing music to seeing colors,” said UC Berkeley vision scientist Stephen Palmer, lead author of a paper published this week in the journal Proceedings of the National Academy of Sciences. [Continue reading…]
New study examines how individuality develops
EurekAlert! reports: The adult brain continues to grow with the challenges that it faces; its changes are linked to the development of personality and behavior. But what is the link between individual experience and brain structure? Why do identical twins not resemble each other perfectly even when they grew up together? To shed light on these questions, the scientists observed forty genetically identical mice that were kept in an enclosure offering a large variety of activity and exploration options.
“The animals were not only genetically identical, they were also living in the same environment,” explains principal investigator Gerd Kempermann, Professor for Genomics of Regeneration, CRTD, and Site Speaker of the DZNE in Dresden. “However, this environment was so rich that each mouse gathered its own individual experiences in it. Over time, the animals therefore increasingly differed in their realm of experience and behavior.”
Each of the mice was equipped with a special micro-chip emitting electromagnetic signals. This allowed the scientists to construct the mice’s movement profiles and to quantify their exploratory behavior. The result: Despite a common environment and identical genes the mice showed highly individualized behavioral patterns. They reacted to their environment differently. In the course of the three-month experiment these differences increased in size.
“Though the animals shared the same life space, they increasingly differed in their activity levels. These differences were associated with differences in the generation of new neurons in the hippocampus, a region of the brain that supports learning and memory,” says Kempermann. “Animals that explored the environment to a greater degree also grew more new neurons than animals that were more passive.”
Adult neurogenesis, that is, the generation of new neurons in the hippocampus, allows the brain to react to new information flexibly. With this study, the authors show for the first time that personal experiences and ensuing behavior contribute to the “individualization of the brain.” The individualization they observed cannot be reduced to differences in environment or genetic makeup.
“Adult neurogenesis also occurs in the hippocampus of humans,” says Kempermann. “Hence we assume that we have tracked down a neurobiological foundation for individuality that also applies to humans.” [Continue reading…]
Neurocriminology: Inside the criminal mind
Adrian Raine writes: The scientific study of crime got its start on a cold, gray November morning in 1871, on the east coast of Italy. Cesare Lombroso, a psychiatrist and prison doctor at an asylum for the criminally insane, was performing a routine autopsy on an infamous Calabrian brigand named Giuseppe Villella. Lombroso found an unusual indentation at the base of Villella’s skull. From this singular observation, he would go on to become the founding father of modern criminology.
Lombroso’s controversial theory had two key points: that crime originated in large measure from deformities of the brain and that criminals were an evolutionary throwback to more primitive species. Criminals, he believed, could be identified on the basis of physical characteristics, such as a large jaw and a sloping forehead. Based on his measurements of such traits, Lombroso created an evolutionary hierarchy, with Northern Italians and Jews at the top and Southern Italians (like Villella), along with Bolivians and Peruvians, at the bottom.
These beliefs, based partly on pseudoscientific phrenological theories about the shape and size of the human head, flourished throughout Europe in the late 19th and early 20th centuries. Lombroso was Jewish and a celebrated intellectual in his day, but the theory he spawned turned out to be socially and scientifically disastrous, not least by encouraging early-20th-century ideas about which human beings were and were not fit to reproduce—or to live at all.
The racial side of Lombroso’s theory fell into justifiable disrepute after the horrors of World War II, but his emphasis on physiology and brain traits has proved to be prescient. Modern-day scientists have now developed a far more compelling argument for the genetic and neurological components of criminal behavior. They have uncovered, quite literally, the anatomy of violence, at a time when many of us are preoccupied by the persistence of violent outrages in our midst.
The field of neurocriminology—using neuroscience to understand and prevent crime—is revolutionizing our understanding of what drives “bad” behavior. More than 100 studies of twins and adopted children have confirmed that about half of the variance in aggressive and antisocial behavior can be attributed to genetics. Other research has begun to pinpoint which specific genes promote such behavior.
Brain-imaging techniques are identifying physical deformations and functional abnormalities that predispose some individuals to violence. In one recent study, brain scans correctly predicted which inmates in a New Mexico prison were most likely to commit another crime after release. Nor is the story exclusively genetic: A poor environment can change the early brain and make for antisocial behavior later in life.
Most people are still deeply uncomfortable with the implications of neurocriminology. Conservatives worry that acknowledging biological risk factors for violence will result in a society that takes a soft approach to crime, holding no one accountable for his or her actions. Liberals abhor the potential use of biology to stigmatize ostensibly innocent individuals. Both sides fear any seeming effort to erode the idea of human agency and free will. [Continue reading…]
Reliability of neuroscience research questioned
Bristol University: New research has questioned the reliability of neuroscience studies, saying that conclusions could be misleading due to small sample sizes.
A team led by academics from the University of Bristol reviewed 48 articles on neuroscience meta-analysis which were published in 2011 and concluded that most had an average power of around 20 per cent – a finding which means the chance of the average study discovering the effect being investigated is only one in five.
The paper, being published in Nature Reviews Neuroscience today [10 April], reveals that small, low-powered studies are ‘endemic’ in neuroscience, producing unreliable research which is inefficient and wasteful.
It focuses on how low statistical power – caused by low sample size of studies, small effects being investigated, or both – can be misleading and produce more false scientific claims than high-powered studies.
It also illustrates how low power reduces a study’s ability to detect any effects and shows that when discoveries are claimed, they are more likely to be false or misleading.
Obama’s big BRAIN project
Kas Thomas writes: President Obama’s kickoff of the BRAIN initiative was a major news item the other day. Widely lauded as the kind of program that can keep America at the forefront of science, Brain Research through Advancing Innovative Neurotechnologies (or, if you prefer, Big Ridiculous Acronyms Inspired by Nonsense) was compared to the Apollo moon program, which “gave us CAT scans” (the President said) and to the Human Genome Project, which altered economic reality as we know it by creating $140 in return for every dollar invested.
The President hailed BRAIN as “a bold new research effort to revolutionize our understanding of the human mind and uncover new ways to treat, prevent, and cure brain disorders like Alzheimer’s, schizophrenia, autism, epilepsy, and traumatic brain injury.”
And for all this great knowledge, we’re going to pay just $100 million (first year).
Thomas goes on to spell out why that amount is nothing more than pocket change, but small as the funding is, it also seems worth noting that half of it is going to DARPA — the Defense Advanced Research Projects Agency, the research wing of the Pentagon.
“DARPA hopes to build tools that can view, measure and control the brain from the cellular and neuronal to the macroscopic levels,” reports the Army Times.
Dystopian fiction not far from becoming security state reality
Nature: In a twist that evokes the dystopian science fiction of writer Philip K. Dick, neuroscientists have found a way to predict whether convicted felons are likely to commit crimes again from looking at their brain scans. Convicts showing low activity in a brain region associated with decision-making and action are more likely to be arrested again, and sooner.
Kent Kiehl, a neuroscientist at the non-profit Mind Research Network in Albuquerque, New Mexico, and his collaborators studied a group of 96 male prisoners just before their release. The researchers used functional magnetic resonance imaging (fMRI) to scan the prisoners’ brains during computer tasks in which subjects had to make quick decisions and inhibit impulsive reactions.
The scans focused on activity in a section of the anterior cingulate cortex (ACC), a small region in the front of the brain involved in motor control and executive functioning. The researchers then followed the ex-convicts for four years to see how they fared.
Among the subjects of the study, men who had lower ACC activity during the quick-decision tasks were more likely to be arrested again after getting out of prison, even after the researchers accounted for other risk factors such as age, drug and alcohol abuse and psychopathic traits. Men who were in the lower half of the ACC activity ranking had a 2.6-fold higher rate of rearrest for all crimes and a 4.3-fold higher rate for nonviolent crimes. The results are published today in the Proceedings of the National Academy of Sciences1.
There is growing interest in using neuroimaging to predict specific behaviour, says Tor Wager, a neuroscientist at the University of Colorado in Boulder. He says that studies such as this one, which tie brain imaging to concrete clinical outcomes, “provide a new and so far very promising way” to find patterns of brain activity that have broader implications for society.
But the authors themselves stress that much more work is needed to prove that the technique is reliable and consistent, and that it is likely to flag only the truly high-risk felons and leave the low-risk ones alone. “This isn’t ready for prime time,” says Kiehl.
Wager adds that the part of the ACC examined in this study “is one of the most frequently activated areas in the human brain across all kinds of tasks and psychological states”. Low ACC activity could have a variety of causes — impulsivity, caffeine use, vascular health, low motivation or better neural efficiency — and not all of these are necessarily related to criminal behaviour. [Continue reading…]
The neuroscience of finding your lost keys
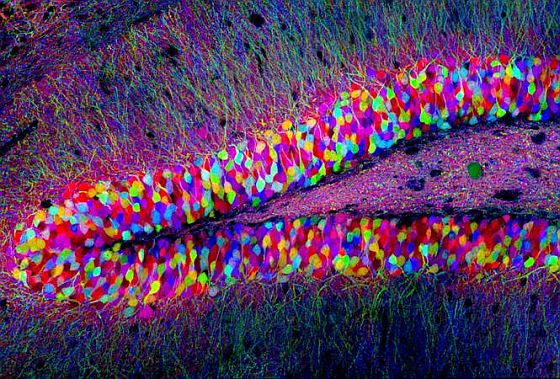
Individual neurons of the dentate gyrus in a 'Brainbow' mouse.
Salk Institute: Ever find yourself racking your brain on a Monday morning to remember where you put your car keys?
When you do find those keys, you can thank the hippocampus, a brain region responsible for storing and retrieving memories of different environments-such as that room where your keys were hiding in an unusual spot.
Now, scientists have helped explain how the brain keeps track of the incredibly rich and complex environments people navigate on a daily basis. They discovered how the dentate gyrus, a subregion of the hippocampus, helps keep memories of similar events and environments separate, a finding they reported March 20 in eLife. The findings, which clarify how the brain stores and distinguishes between memories, may also help identify how neurodegenerative diseases, such as Alzheimer’s disease, rob people of these abilities.
“Everyday, we have to remember subtle differences between how things are today, versus how they were yesterday – from where we parked our car to where we left our cellphone,” says Fred H. Gage, senior author on the paper and the Vi and John Adler Chair for Research on Age-Related Neurodegenerative Disease at Salk. “We found how the brain makes these distinctions, by storing separate ‘recordings’ of each environment in the dentate gyrus.”
The process of taking complex memories and converting them into representations that are less easily confused is known as pattern separation. Computational models of brain function suggest that the dentate gyrus helps us perform pattern separation of memories by activating different groups of neurons when an animal is in different environments.
However, previous laboratory studies found that in fact the same populations of neurons in the dentate gyrus are active in different environments, and that the way the cells distinguished new surroundings was by changing the rate at which they sent electrical impulses. This discrepancy between theoretical predictions and laboratory findings has perplexed neuroscientists and obscured our understanding of memory formation and retrieval. [Continue reading…]
Mind control in the name of medicine
Is it possible to connect two brains in such a way that the thoughts in one could control those in the other?
That sounds like a question whose answer would have interested Hitler and Stalin. Wouldn’t this be every dictator’s dream: not only the capacity to control how others behave but also how they think?
On the other hand, if you’re a research neuroscientist and you want to conduct experiments in mind control, all you need do to allay public fears is to say that what you hope to discover is going to help paralyzed people walk again. Whenever there’s a hint of Dr Frankenstein, it’s always good to invoke Jesus.
The New York Times reports: In an experiment that sounds straight out of a science fiction movie, a Duke neuroscientist has connected the brains of two rats in such a way that when one moves to press a lever, the other one does, too — most of the time.
The neuroscientist, Miguel Nicolelis, known for successfully demonstrating brain-machine connections, like the one in which a monkey controlled a robotic arm with its thoughts, said this was the first time one animal’s brain had been linked to another.
The question, he said, was: “Could we fool the brain? Could we make the brain process signals from another body?” The answer, he said, was yes.
He and other scientists at Duke, and in Brazil, published the results of the experiment in the journal Scientific Reports. The work received mixed reviews from other scientists, ranging from “amazing” to “very simplistic.”
Much of Dr. Nicolelis’s work is directed toward creating a full exoskeleton that a paralyzed person could operate with brain signals. Although this experiment is not directly related, he said, it helps refine the ability to read and translate brain signals, an important part of all prosthetic devices connected to the brain, and an area in which brain science is making great advances.
He also speculated about the future possibility of a biological computer, in which numerous brains are connected, and views this as a small step in that direction.
Songbirds’ brains coordinate singing with intricate timing
University of Chicago: As a bird sings, some neurons in its brain prepare to make the next sounds while others are synchronized with the current notes—a coordination of physical actions and brain activity that is needed to produce complex movements, new research at the University of Chicago shows.
In an article in the current issue of Nature, neuroscientist Daniel Margoliash and colleagues show, for the first time, how the brain is organized to govern skilled performance—a finding that may lead to new ways of understanding human speech production.
The new study shows that birds’ physical movements actually are made up of a multitude of smaller actions. “It is amazing that such small units of movements are encoded, and so precisely, at the level of the forebrain,” said Margoliash, a professor of organismal biology and anatomy and psychology at UChicago.
“This work provides new insight into how the physics of controlling vocal signals are represented in the brain to control vocalizations,” said Howard Nusbaum, a professor of psychology at UChicago and an expert on speech.
By decoding the neural representation of communication, Nusbaum explained, the research may shed light on speech problems such as stuttering or aphasia (a disorder following a stroke). And it offers an unusual window into how the brain and body carry out other kinds of complex movement, from throwing a ball to doing a backflip.
“A big question in muscle control is how the motor system organizes the dynamics of movement,” said Margoliash. Movements like reaching or grasping are difficult to study because they entail many variables, such as the angles of the shoulder, elbow, wrist and fingers; the forces of many muscles; and how these change over time,” he said.
How flies fly and what makes flies smarter than people
Evolution, viewed as a process of progression (which it isn’t) leads to the notion that as the possessors of the most complex brains, we sit proudly at the top of the evolutionary pyramid. Even if one doesn’t question that it makes sense to assign ourselves this position, there’s no disputing that our tenure has thus far been very brief.
Flies on the other hand, have been around for about 200 million years and as Michael Dickinson explains, this has a lot to do with the sophistication of their neurology — which is to say, their ability to do much more with much less.
Human cognition depends upon slow-firing neurons
YaleNews: Good mental health and clear thinking depend upon our ability to store and manipulate thoughts on a sort of “mental sketch pad.” In a new study, Yale School of Medicine researchers describe the molecular basis of this ability — the hallmark of human cognition — and describe how a breakdown of the system contributes to diseases such as schizophrenia and Alzheimer’s disease.
“Insults to these highly evolved cortical circuits impair the ability to create and maintain our mental representations of the world, which is the basis of higher cognition,” said Amy Arnsten, professor of neurobiology and senior author of the paper published in the Feb. 20 issue of the journal Neuron.
High-order thinking depends upon our ability to generate mental representations in our brains without any sensory stimulation from the environment. These cognitive abilities arise from highly evolved circuits in the prefrontal cortex. Mathematical models by former Yale neurobiologist Xiao-Jing Wang, now of New York University, predicted that in order to maintain these visual representations the prefrontal cortex must rely on a family of receptors that allow for slow, steady firing of neurons. The Yale scientists show that NMDA-NR2B receptors involved in glutamate signaling regulate this neuronal firing. These receptors, studied at Yale for more than a decade, are responsible for activity of highly evolved brain circuits found especially in primates.
The disease-bias of medical research dictates that research funding is invariably going to hinge on the promise of treatment for one or more major disorders, in this case schizophrenia and Alzheimer’s. Still, in research such as that described above, it might be just as interesting and fruitful to investigate the neurological impact of what have become ubiquitous forms of behavior such as text-messaging.
In non-neurological language the use of these slow-firing neurons seems to include a constellation of cognitive activities including deliberation, reflection, analysis, and problem-solving.
Do handheld devices and the distractions they cause impair our ability to create and maintain sound mental representations of the world?
I don’t imagine Apple or anyone else with a vested interest in proving that hyper-connectivity is harmless, would welcome this line of inquiry, but still, it seems like a question worth asking.
Neurons gone wild
Daniel Dennett says: Each neuron is imprisoned in your brain. I now think of these as cells within cells, as cells within prison cells. Realize that every neuron in your brain, every human cell in your body (leaving aside all the symbionts [such as mitochondria]), is a direct descendent of eukaryotic cells that lived and fended for themselves for about a billion years as free-swimming, free-living little agents. They fended for themselves, and they survived.
They had to develop an awful lot of know-how, a lot of talent, a lot of self-protective talent to do that. When they joined forces into multi-cellular creatures, they gave up a lot of that. They became, in effect, domesticated. They became part of larger, more monolithic organizations. My hunch is that that’s true in general. We don’t have to worry about our muscle cells rebelling against us, or anything like that. When they do, we call it cancer, but in the brain I think that (and this is my wild idea) maybe only in one species, us, and maybe only in the obviously more volatile parts of the brain, the cortical areas, some little switch has been thrown in the genetics that, in effect, makes our neurons a little bit feral, a little bit like what happens when you let sheep or pigs go feral, and they recover their wild talents very fast.
Maybe a lot of the neurons in our brains are not just capable but, if you like, motivated to be more adventurous, more exploratory or risky in the way they comport themselves, in the way they live their lives. They’re struggling amongst themselves with each other for influence, just for staying alive, and there’s competition going on between individual neurons. As soon as that happens, you have room for cooperation to create alliances, and I suspect that a more free-wheeling, anarchic organization is the secret of our greater capacities of creativity, imagination, thinking outside the box and all that, and the price we pay for it is our susceptibility to obsessions, mental illnesses, delusions and smaller problems.
We got risky brains that are much riskier than the brains of other mammals even, even more risky than the brains of chimpanzees, and that this could be partly a matter of a few simple mutations in control genes that release some of the innate competitive talent that is still there in the genomes of the individual neurons. But I don’t think that genetics is the level to explain this. You need culture to explain it.
This, I speculate, is a response to our invention of culture; culture creates a whole new biosphere, in effect, a whole new cultural sphere of activity where there’s opportunities that don’t exist for any other brain tissues in any other creatures, and that this exploration of this space of cultural possibility is what we need to do to explain how the mind works. [Continue reading…]
Measuring consciousness
In a feature article for the Atlantic, Joshua Lang explores the phenomenon of anesthesia awareness: where patients undergoing surgery are conscious yet paralyzed and thus unable to signal the pain and terror they are experiencing.
On a warm afternoon in Madison, Wisconsin, last spring, a psychiatrist was pointing an electromagnetic gun at my brain.
“Put your arm in your lap,” he said.
I obeyed. My head was dressed in a 60-electrode, high-density EEG-recording device. The doctor stood behind my chair, eyeing a digitized MRI of my brain and gliding the gun over my scalp until he found his target: my motor cortex.
“Relax.”
I tried.
The gun clicked. My forehead muscles twitched. My arm leapt out of my lap, straight into the air, as if yanked by invisible puppet strings. “Do it again,” I said.
This process is called transcranial magnet stimulation, or TMS. It is the key to a device that Giulio Tononi, one of the most-talked-about figures in anesthesiology since Nassib Chamoun, hopes will provide a truly comprehensive assessment of consciousness. If successful, Tononi’s device could reliably prevent anesthesia awareness. But his ambitions are much grander than that. Tononi is unraveling the mystery of consciousness: how it works, how to measure it, how to control it, and, possibly, how to create it.
At the heart of Tononi’s work is his integrated-information theory, which is based on two distinct principles, as intuitive as they are scientific. First, consciousness is informative. Every waking moment of your life provides a nearly infinite reservoir of possible experiences, each one different from the next. Second, consciousness is integrated: you can’t process this information in parts. When you see a red ball, you can’t experience the color red separately from the shape of the ball. When you hear a word, you can’t experience the sound of it separately from its meaning. According to this theory, a more conscious brain is both more informative (it has a deeper reservoir of experiences and stimuli) and more integrated (its perception of these experiences is more unified) than a less conscious one.
Compare the brain to New York City: just as cars navigate the city’s neighborhoods via a patchwork of streets, bridges, tunnels, and highways, electrical signals traverse the brain via a meshwork of neurons. Tononi’s theory predicts that in a fully conscious brain, traffic in one neighborhood will affect traffic in other neighborhoods, but that as consciousness fades—for instance, during sleep or anesthesia—this ripple effect will decrease or disappear.
In 2008, in one of several experiments demonstrating this effect, Tononi pulsed the brains of 10 fully conscious subjects with his electromagnetic gun—the equivalent of, say, injecting a flood of new cars into SoHo. The traffic (the electromagnetic waves) rippled across Manhattan (the brain): things jammed up in Tribeca and Greenwich Village, even in Chelsea. Tononi’s EEG electrodes captured ripples and reverberations that were different for every subject and for every region of the brain, patterns as complex and varied as the traffic in Manhattan on any given day.
Tononi then put the same subjects under anesthesia. Before he pulsed his gun again, the subjects’ brain traffic seemed as busy as when they were conscious: cars still circulated in SoHo and Tribeca, in Greenwich Village and Chelsea. But the pulse had a drastically different effect: This time, the traffic jam was confined to SoHo. No more ripples. “It’s as if [the brain] has fragmented into pieces,” Tononi told me. He published these findings in 2010, and also used them to file a patent for “a method for assessing anesthetization.”
It seems emblematic of the contemporary bio-medical approach to understanding human experience, that in attempting to understand the nature of consciousness one of the most valuable outcomes might be a method for verifying unconsciousness.
How our brains categorize and map everything we see
ExtremeTech: If not for our brains, our eyes wouldn’t be able to process anything. Considering how much time we spend with our eyes open, our brains are constantly dealing with an influx of visual data. The brain needs to store that data somewhere, and thanks to scientists over at the University of California, Berkeley, we now have our first map of not only where the brain puts all that information, but how our grey matter organizes it.
The study (PDF), led by neuroscience doctoral student Alexander Huth, had five participants watch two hours of movie trailers that contained over 1,700 categories of actions and objects. During that time, their brain activity was recorded using functional Magnetic Resonance Imaging (fMRI), measuring blood flow in various spots in the brain. Using linear regression, the scientists were then able to analyze the collected data, and subsequently build a model showing how all of those actions and objects fit into around 30,000 locations within the cortex.
After that point the researchers translated the model to a visual form. Using principal component analysis – a mathematical procedure used to provide a synopsis for a large amount of data — the scientists were able to visualize those 1,700 categories and how they related to one another, creating the chart shown to the right.
The map is made [of] “semantic neighborhoods,” which are essentially just categories of things that the brain finds similar to each other. The researchers found that, for instance, the brain organizes the categories of “humans” and “animals” in a related manner, whereas “eyeball” and “car” are stored in completely different areas of the brain. Along with finding out how the brain organizes different categories of objects, the researchers also found out that different people’s brains organize things in similar ways. [Continue reading…]
The graphic above is not a representation of a neural network. It’s simply a visual representation of the WordNet semantic network from which the 1,700 categories used in this research were derived.